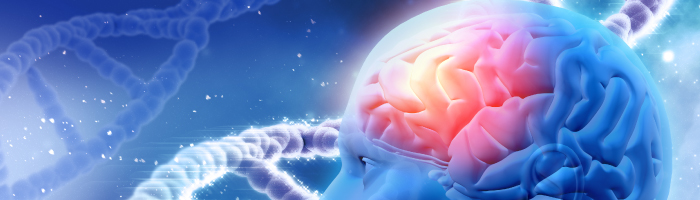
This post is the third of a three part blog series. Click here to read Part 1 and Part 2.
In our previous post, we discussed the approaches to help you identify, enroll and retain rare disease populations in clinical trials. Once the right patients have been enrolled in the trial, the challenge becomes measuring the efficacy of the experimental treatment and determining whether it’s producing the desired effect. However, invasive drug delivery methods, such as IV injection, can complicate this measurement and establishing whether the therapy actually reaches the target tissue can be difficult.
New Approaches to CNS Therapeutic Delivery and Monitoring
For diseases that affect the CNS, it can be hard to collect tissue samples capable of confirming diagnosis, measuring disease progression or even monitoring the effectiveness of a specific treatment. For example, a tissue biopsy is often used to study samples in oncology, but clinicians are often unable to take a biopsy of the brain or spinal cord, except in the case of a post-mortem.
Imaging, such as molecular PET, has been used to confirm diagnosis of diseases like Alzheimer’s and Parkinson’s, but target limitations and concerns over radiation exposure have limited its use. Cerebrospinal fluid (CSF) has been a more useful way to detect disease markers, but because of its relatively invasive nature, a few considerations must be taken into account before it’s used during a trial. When introducing CSF collection in a clinical trial, it’s important to work with the site to pre-empt any patient or family fear or resistance, or even investigator fear or resistance. Investigators need to use appropriate needles to minimize headache and to attend to factors that may impact the analyses, such as circadian fluctuation in various markers.
Drug development for neurological diseases faces another hurdle: how to get therapies past the blood-brain barrier. While protein, antibody, gene and cell therapies could be effective ways to treat diseases affecting the CNS, even small molecule drugs are often blocked from entering the brain by this barrier, representing a significant challenge in drug delivery.
The blood-brain barrier challenge has prompted some drug developers to seek out other ways of getting their therapeutic to its target. Neurosurgical delivery of therapeutics directly into the brain, and injection into the CNS via the CSF have both been tested, however these methods also have their limitations. For example, Oxford Biomedica’s ProSavin is a gene therapy designed to increase dopamine production in the brains of patients with Parkinson’s disease. While the therapy had a favorable safety profile in clinical trials, it was only moderately efficacious.
Some studies have found that therapies being delivered to the brain through the CSF could be promising to localized targets, but the drug often fails to reach deeper tissue. This is the case with nusinersin, an anti-sense oligonucleotide that modifies pre-messenger RNA splicing of the SMN2 gene, which, in turn, increases production of motor neuron protein in spinal muscular atrophy. Molecular modifications could help drugs cross the blood-brain barrier, even when they’re delivered intravenously. Designing a drug that’s lipid-soluble could help mediate this drug delivery problem, however the effects of active transporter efflux, which can pump drugs back out of the cell, can be hard to overcome. Carriers and transporters are also available that can help even macromolecules reach the brain. In particular, receptor-mediated and absorptive endocytosis may allow otherwise nonpenetrant therapies to reach the brain.
Fusion proteins and the use of CNS trophic viruses as vectors are some promising new approaches in this area of drug development. Both tactics have the potential to lower the traditionally large systemic doses necessary to have a measurable effect, with the ability to edit the promoter sequences of viral vectors and increase their target specificity. Such therapies hold the potential to modify the genome of the target cells, so instead of requiring a lifetime of administration it’s possible to administer just on one occasion. But once the drug delivery method has been optimized, the question becomes, “did it reach the CNS?” Utilizing tools to measure the impact and effectiveness of experimental CNS drugs is also trickier than in other disease areas.
Again, PET labelling can help confirm that the drug has entered the brain and even bound to its target receptor, so this technique is commonly used for psychiatric therapies. While it’s possible for catheters within the brain to sample extracellular fluid, this type of assay is rarely done in human patients because it is highly invasive. While sampling the CSF might not give an accurate estimate of drug concentrations within the brain, it can help assess the level of target engagement for a given therapy. However, the invasive nature of lumbar punctures prevents frequent sampling of CSF.
Perhaps the most important variable to consider is whether the treatment actually improved patient outcomes. Indications with very few available patients need the best possible study designs. This is true for orphan diseases, and again true when subsets of common diseases become orphan. Clinical evaluation of the patient is critical.
When it comes to assessing outcomes in neurological clinical trials, careful rater training and certification are crucial. Patient-reported outcomes also play a key role in this type of trial, particularly if blinding study staff is difficult, as is the case with neurosurgical treatments. These patient-reported outcomes are increasingly being facilitated by personal devices and the ever-present technology that patients use in their everyday lives. Bring-your-own-device has become increasingly common in clinical trials. It alleviates some of the burden of trying to provide devices across large scale trials and increases the patient acceptance of not having to carry multiple devices around. Home monitoring devices – including wearable sensors and home video systems – can act as an objective supplement to the patient’s own responses.
Ultimately, the goal of these rare disease clinical trials is to gather the most data from the often small number of enrolled participants, while ensuring that the burden on patients and sites is minimized. Despite the challenges inherent to rare disease studies, categorizing patients into smaller subsets may aid in the development of the most efficacious therapies.
On-Demand Webinar
Getting Treatment Options to Rare Disease Patients Faster: Putting Patients First in Clinical Trials (Watch Now)